Circularity in Food and Crop Production Systems
- The Circular Collective
- Oct 28, 2020
- 5 min read
Updated: Oct 29, 2020
Global food security has been increasingly threatened in recent times as a result of growing demographics, shifts in consumption patterns especially in urban areas, economic trends and the adverse impacts of climate change. At both global and local levels, crop production may be limited by the availability of water, land, nutritional amenities etc. as well as ecological interactions such as shifts in rainfall patterns and precipitation levels, temperature fluctuations, and natural disasters and this restricts food production levels to keep up with the growing demand (FAO, 2012).
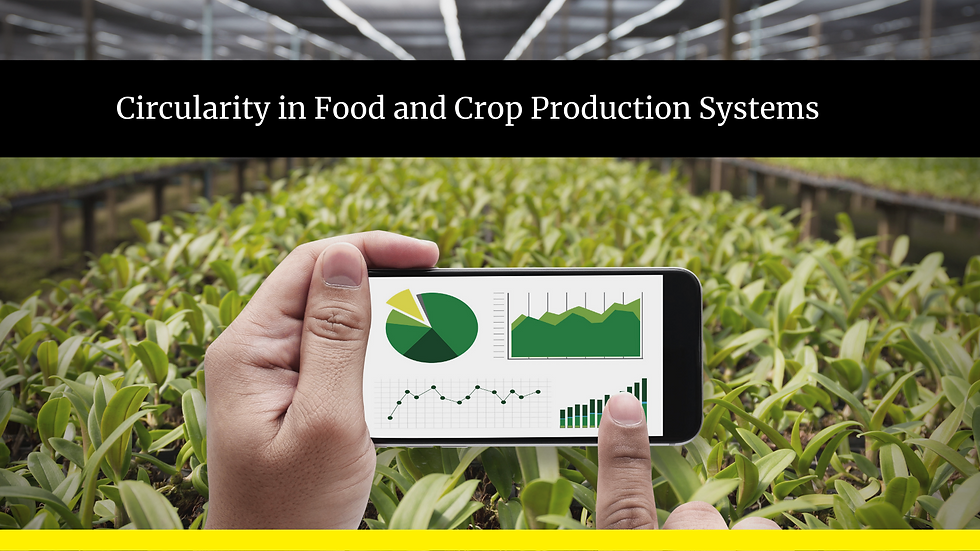
Circularity, as a principle, aims to mitigate the impact of climate change and other aforementioned factors untowardly impacting crop production and maximising utilisation of the biomass of crops produced. Hence, circularity in crop production exists at multiple levels, targeting the yield of crops in a given location and subsequently increasing the functionality of the cultivar produced.
Implementing circularity in pre-existing frameworks with negative impacts on the environment provides a robust approach to shift to closed-loop systems with minimum waste and carbon footprints (Tsenga, et al., 2019). Food supply chains face high losses due to the perishable nature of food hence contriving circularity in the same results in fewer losses, aid in balancing demand and supply levels as well as reduce greenhouse gas emissions at each stage of the supply chain (Foong, et al., 2019).
Tackling the Yield Gap
One of the critical tenets of circularity in food and crop production is recognising the presence of a ‘yield gap’ and further undertaking steps to reduce the same while concurrently focusing efforts towards increasing the maximum ‘potential yield’ in a given location. A yield gap refers to the difference between said potential yield or the amount of cultivar than can be produced given no limitation to factors such as water, fertility etc. and the absence of yield-reducing factors such as pests, weeds etc., and the ‘actual yield’ or the amount of cultivar produced in a given location by farmers (de Boer & van Ittersum, 2018). While yield gaps are ordinarily 80 to 85 per cent of the maximum theoretical potential and this share is both environmentally and economically feasible, in many countries around the globe this figure can drop to 50 per cent, even 20 in some cases, which reflect low productivity levels and could result in potentially catastrophic drops in supply levels over the coming decades (de Boer & van Ittersum, 2018).
The basal step to tackling such a yield gap is to identify and acknowledge the impact of yield-defining, yield-limiting and yield-reducing factors on agriculture. Yield-defining factors include climatic conditions and crop genetics, limiting factors include the availability of water, land and other resources, and reducing factors include the occurrence of weeds, pests and diseases.
The potential yield of crops in a location can be increased by improving radiation capture, increasing efficiency of converting radiation to biomass and increasing the share of harvested biomass, to appropriately take advantage of pre-existing ecological elements like sunlight. Magnifying this exploitation of radiation is a key feature of circularity in crop production as it aligns with the rudimentary proposition of minimising value loss of given resources. This can be achieved by adding a ground cover of leaves, extending crop growth durations, to an extent, and early crop establishment to increase radiation capture. However, a balance is to be drawn with regards to crop growth durations as while it might lead to increased biomass of cultivar produced, it might not result in higher yields and maximise the efficiency of crop growth. Efforts in plant breeding are presently focused towards improving the quality of the main product while investment towards increasing the utility value of by-products ties in better with circular principles and maximises yield per unit of biomass as well.
Recycling in Food Systems
Apart from the direct products of food production, there are numerous by-products as well including crop residues like beet pulp, and co-products including but not limited to food waste and excreta. To implement circularity, recycling must be instigated to appropriately utilise such entrails by re-introducing products not suitable for human consumption to the food production process. This can be achieved efficiently as such by-products contain nutrients like phosphorus and nitrogen, and carbon which can be converted to structural material, fertiliser, fuel or fodder. Hence, recycling is involved in improving soil quality, providing inexpensive fodder for animal breeding, creating biofuel to reduce greenhouse gas emissions, and increasing levels of carbon sequestration in the soil.
Challenges to Circularity in Crop Production
While the aforementioned benefits of circularity in food production act as incentives to implement the same, there are many barriers to its implementation as well. Recycling food waste and by-products have the con of potentially being unhygienic and hence transmitting diseases if used as animal fodder, especially if said waste contains animal residues. As a result, the European Union and many South Asian countries have placed regulations surrounding the use of food waste in livestock breeding (Zu Ermgassen, et al., 2016). Experts have also criticised the footprint approach to circularity in food production where the focus is towards minimising waste and carbon footprints to tackle environmental challenges while evading the complex inter-linkages in food cycles as well as the feed-fuel-food competition issue (Dixon, et al., 2010).
Despite the above barriers to a circular approach to food supply chains, such a concept put into practice can have immensely positive impacts not only on the environment and climate change mitigation efforts but also on food production systems by increasing their productivity and efficiency and creating a better balance between supply and demand levels. Hence, in light of growing concerns about global food security, circularity provides a ready avenue to explore at a global scale.
About the Author
Shreyas Ashok Kumar is an undergraduate student doing MBA(Tech.) in Chemical Engineering with a passion to work for Sustainability and Circular Economy. His mission is to educate people about Sustainability, Climate Change, Circular Economy & Water Accountability. Saravanakumaran wishes to make each household in India water sufficient, sustainable and zero-waster.
References:
Boer, I. J., & Ittersum, M. K. (2018). Circularity in agricultural production. Wageningen University and Research.
de Ponti, T., Rijk, B., & van Ittersum, M. K. (2012). The crop yield gap between organic and conventional agriculture. Agricultural Systems, 1-9.
de Vries, M., van Middelaar, C., & de Boer, I. (2015). Comparing environmental impacts of beef production systems: A review of life cycle assessments. Livestock Science, 279-288.
Dixon, J., Li, X., Msangi, S., Amede, T., Bossio, D., Ceballos, H., . . . H. (2010). Feed, food and fuel: Competition and potential impacts on small-scale crop–livestock–energy farming systems. Addis Ababa: International Livestock Research Institute (ILRI).
Ermgassen, E. K., Phalan, B., Green, R. E., & Balmford, A. (2016). Reducing the land use of EU pork production: where there’s swill, there’s a way. Food Policy Vol. 58, 35-48.
FAO. (2012). World agriculture towards 2030/2050: the 2012 revision. Food and Agriculture Organization of the United Nations.
Foong, S. Z., Goh, C. K., Supramaniam, C. V., & S.Ng, D. K. (2019). Input–output optimisation model for sustainable oil palm plantation development. Sustainable Production and Consumption, 31-46.
Ponisio LC, M’Gonigle LK, Mace KC, Palomino J, de Valpine P, Kremen C. 2015 Diversification practices reduce organic to conventional yield gap. Proc. R. Soc. B 282: 20141396.
Tsenga, M.-L., Chiub, A. S., Chien, C.-F., & Tan, R. R. (2019). Pathways and barriers to circularity in food systems. Resources, Conservation & Recycling 143, 236-237.
van Ittersum, M. K., Cassman, K. G., Grassini, P., Wolf, J., Tittonell, P., & Hochman, Z. (2013). Yield gap analysis with local to global relevance—A review. Field Crops Research, 4-17.
Van Zanten, H. H., Van Ittersum, M. K., & Boer, I. J. (2019). The role of farm animals in a circular food system. Global Food Security Vol. 21, 18-22.
Comments